News and Insights
Explore the EdenTree Investment News and Insights page, offering timely and informative content to navigate the dynamic world of investments and make informed decisions.
Opinion
What the blackouts in Spain and Portugal could mean for green infrastructure investment
Recent blackouts in Spain and Portugal have exposed weaknesses in the region’s electricity networks. This has the potential to accelerate investment in grid modernisation and utility-scale battery storage, presenting long-term investment opportunities for sustainable infrastructure investors, writes Tommy Kristoffersen, Green Infrastructure Fund Manager at EdenTree.
read moreFilter by
Loading
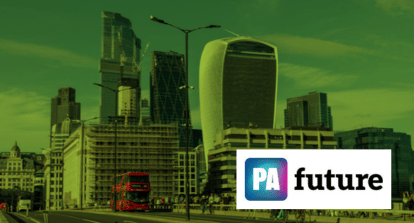
In the News 30 Apr 2025
Regulation and political polarisation: Why one step back could mean two steps forward
read more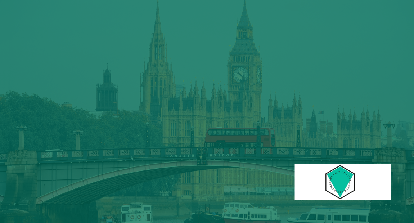
In the News 22 Apr 2025
ACT Signatory Journey: ‘Collaborative engagement is key to wider progress’
read more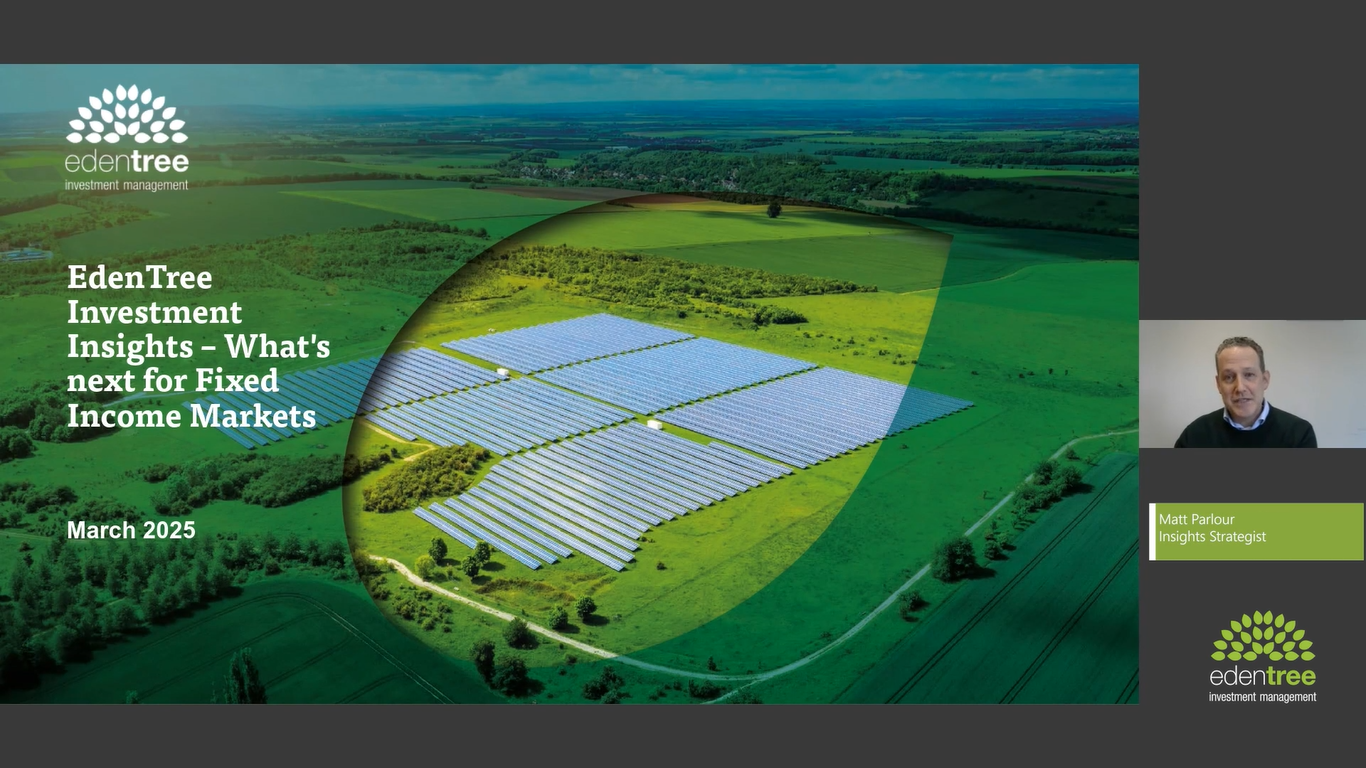
Video 11 Mar 2025
Webinar: EdenTree Investment Insights – What’s next for Fixed Income Markets?
read more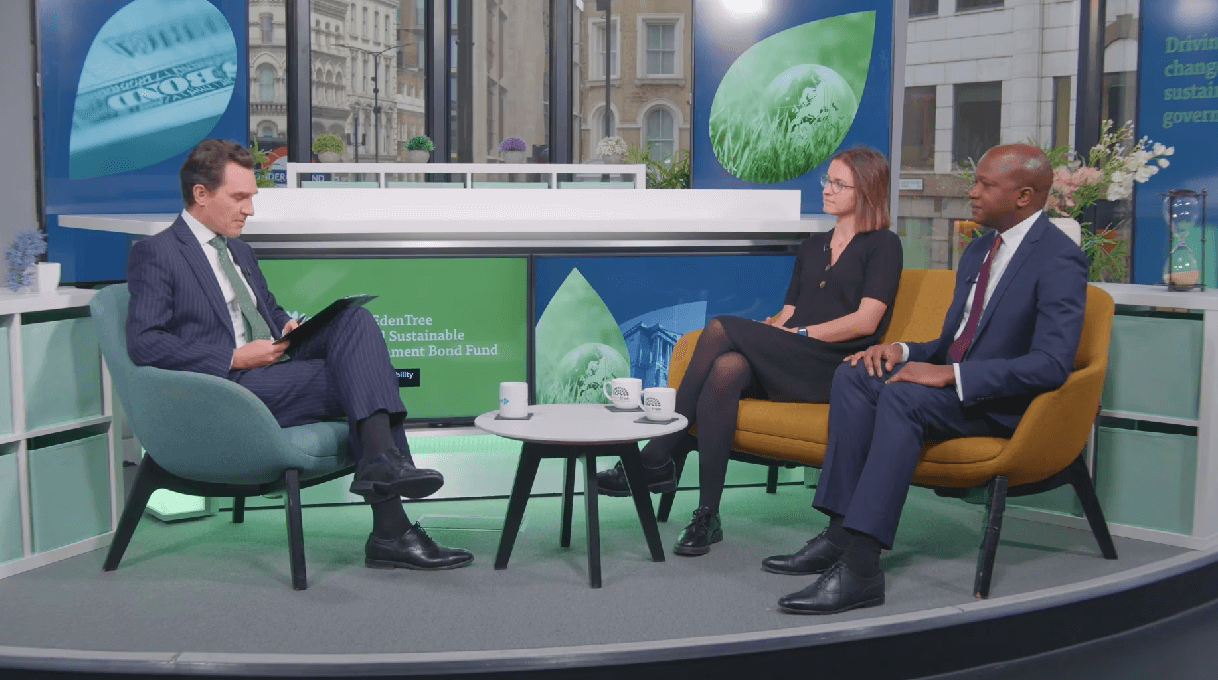
Video 19 Feb 2025
EdenTree’s Global Sustainable Government Bond Fund – our unique solution to the market
read more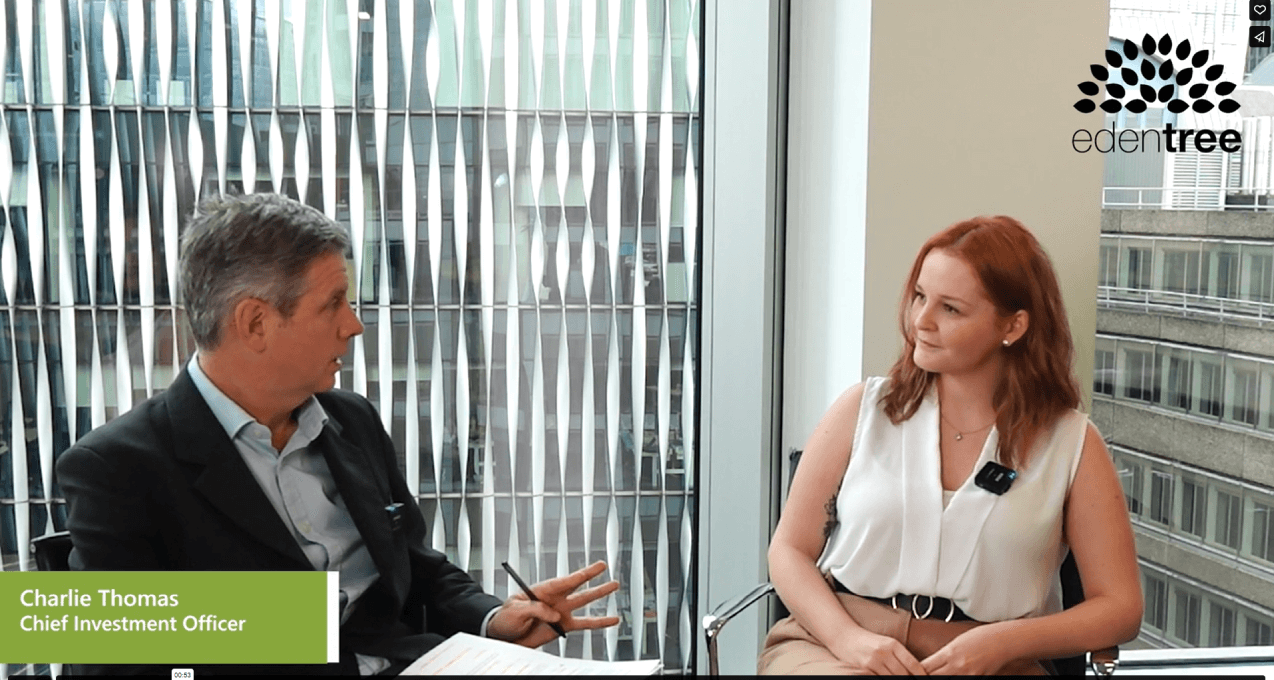
Video 20 Jan 2025