News and Insights
Explore the EdenTree Investment News and Insights page, offering timely and informative content to navigate the dynamic world of investments and make informed decisions.
Video
EdenTree’s Global Sustainable Government Bond Fund – our unique solution to the market
In this video with Asset TV, David Katimbo-Mugwanya and Carlota Esguevillas discuss the demand for the Global Sustainable Government Bond Fund and what makes it unique for investors.
read moreFilter by
Loading
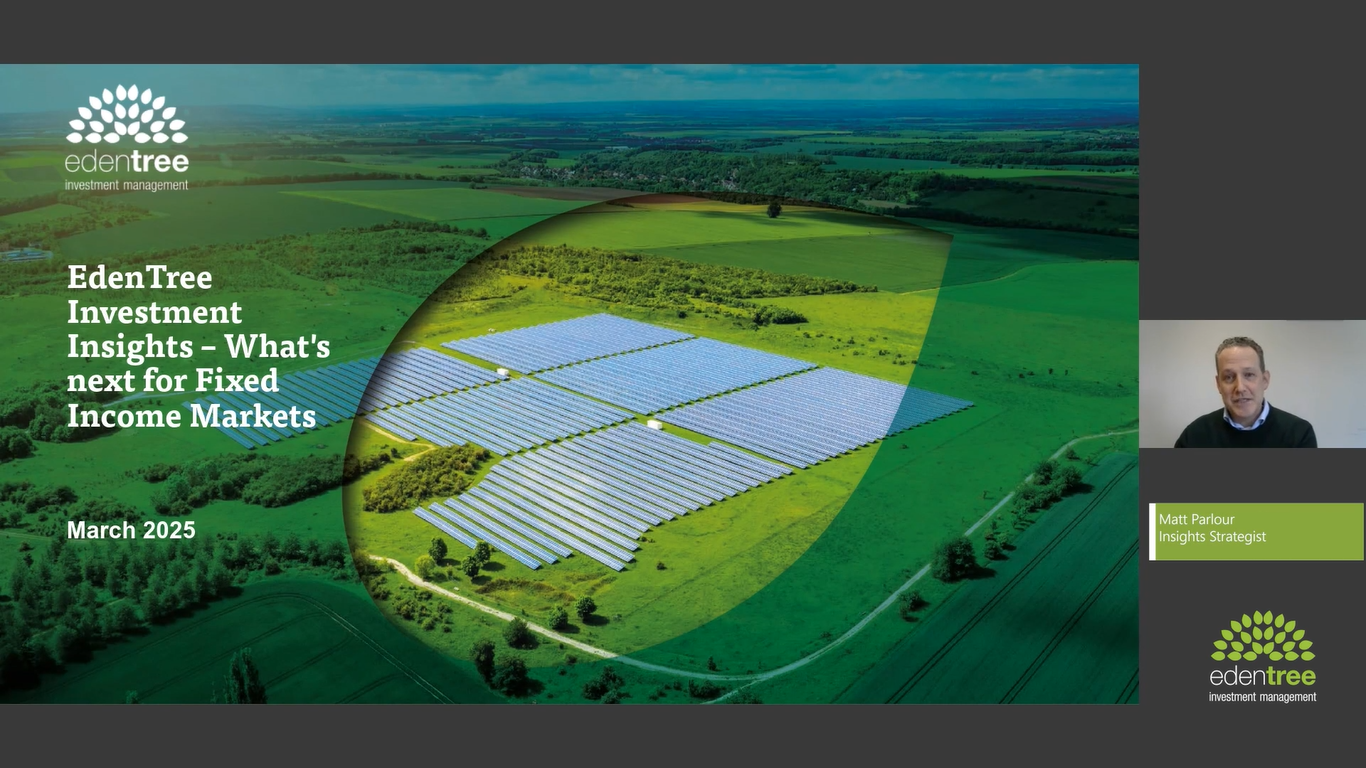
Video 11 Mar 2025
Webinar: EdenTree Investment Insights – What’s next for Fixed Income Markets?
read more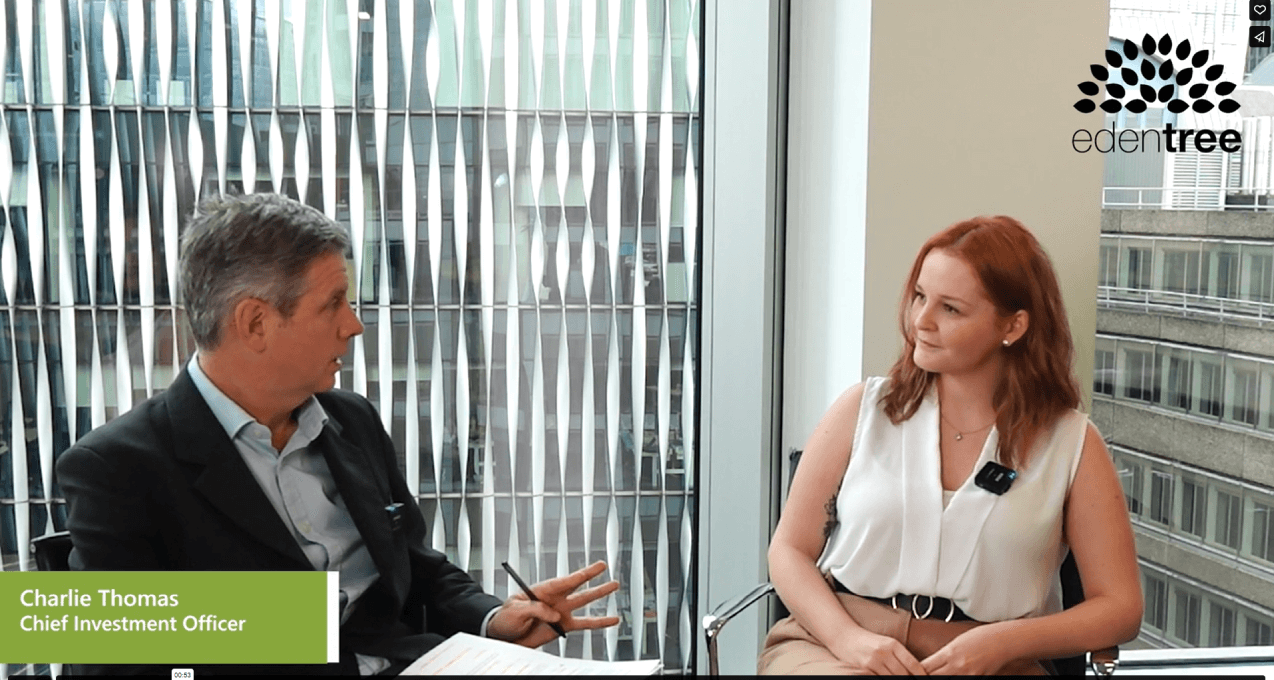
Video 20 Jan 2025
Navigating a Green Future: What are the challenges and opportunities amid the US election results?
read more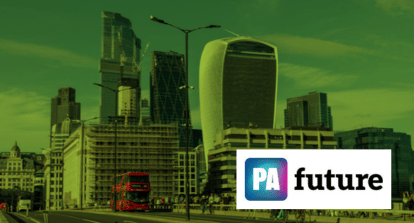
In the News 09 Jan 2025